- FMA
- The Fabricator
- FABTECH
- Canadian Metalworking
Turn To New Technology
Breaking chips is key when machining automotive transmission components
- By Kevin Burton
- July 13, 2016
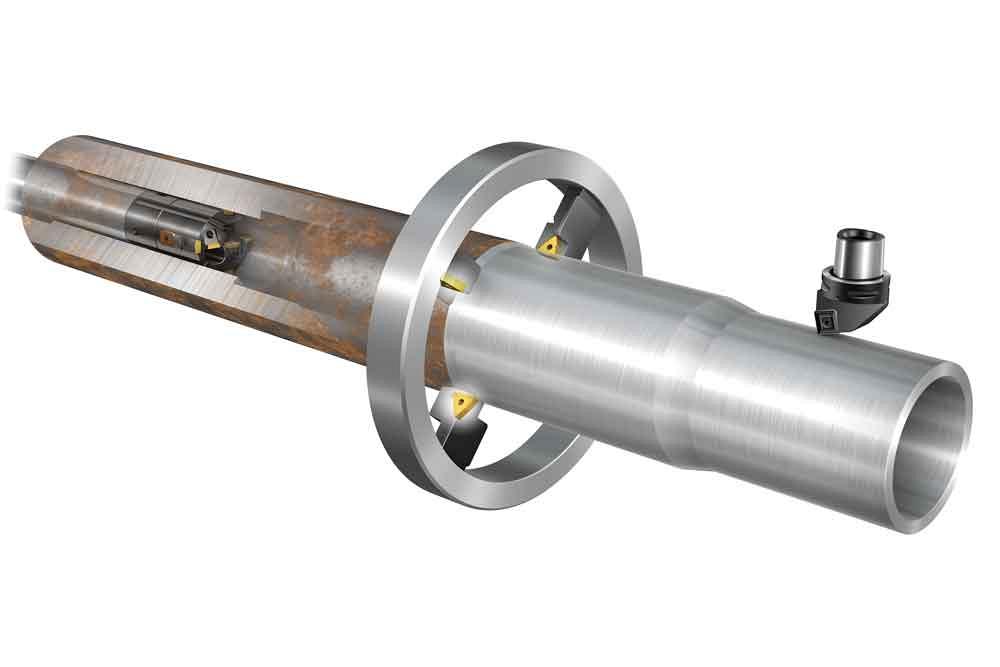
Selecting the proper insert nose radius, DOC, feed rate, and insert geometry will create small, easily handled chips.
Chip control is the bane of every shop’s existence, and knowing how to consistently break chips in ductile steels like SAE 1018, 1020, and 8620 is the holy grail of the tooling industry.
When a shop experiences chip control issues, it affects their bottom line through machine downtime, scrapped or reworked components, lost inventory caused by broken tools, and even employee injury.
Chip control issues typically are found in semifinishing, finishing, and variable-DOC applications, which are the majority of turning applications in the automotive transmission industry.
Despite the technological advances in the tooling industry, machining these ductile materials still causes headaches for operators, engineers, and production managers. With the automotive industry anticipating peak production levels, shops need to solve this problem to keep up with demand and stay profitable.
When it comes to solving chip control problems, physics and economics are not on our side. Everything we have been taught about efficient machining, including tools with positive lead angles and using the largest nose radius possible, now works against us. While both of these options thin the chip, which increases tool life and productivity, they also make chip breaking more difficult. In addition to this problem, many components have Ra requirements that necessitate a large nose radius be used to achieve an acceptable surface finish.
The long, stringy chips that are produced when turning ductile steel can wreak havoc on production.
Chips that don’t break properly often wrap around tools, causing downtime and frustrating operators who need to stop the machine frequently to manually detangle and evacuate the chips. Not only does this take time, the edges of the chips are hot and extremely sharp, putting the operator at risk for injury. In addition, chips can drag along the parts, scoring them and leading to scrapped or reworked components. More trouble ensues when long chips become trapped between the cutting edge and the component, resulting in tool breakage because the tool ends up recutting that chip.
In automated operations, cells with material handling robots or inline gauging also are problems because chips can interfere with the performance of robots and give false readings on gauges.
Know Your Parameters
In a mass production environment, the components being machined are castings or forgings. As a result, hogging off material is usually not an issue.
Improving chip control in these operations comes down to four factors:
- Nose radius
- DOC
- Feed rate
- Top form geometry of the insert
The goal is to maximize the chip area as much as possible while maintaining the accuracy of the component and upholding a productive cycle time.
To increase the chip area, DOCs that are less than the nose radius should not be taken. When a DOC is 0.010 in. per side, with an insert that has a 0.031-in. nose radius, it thins the chip by about 60 per cent, making it much more difficult to break.
The old-school rule of thumb is that the DOC per side should never be less than 66 per cent of the nose radius because at that point, all chip thinning stops.
To maximize the feed rate to increase the chip area, the spindle speed needs to be adjusted accordingly. By far, spindle speed is the cutting parameter that most influences tool life. While DOC and feed rate also matter, they have much less of an impact.
Of course, the other rule of thumb is that higher feed rates will sacrifice your surface finish. This is where wipers are best applied. When they were first introduced, wiper inserts were only used as a way to improve the surface finish. However, we now realize that these inserts can also increase productivity by increasing the feed rate while maintaining (and in many cases improving) the surface finish.
Use New Insert Technology
Most turning inserts today have a geometry pressed into the rake face to control chips. With literally hundreds of geometries available, shops can choose the best geometries to tackle different applications and component materials. When it comes to working with ductile steel, these materials require inserts with some very specific characteristics to form proper chips.
In most cases, the edge line -- or primary land -- should be narrow. For one-pass machining in which we have variable DOCs, it is common to use geometries with lands that vary from 0.004 to 0.010 in.
The key here is to make sure your feed rate exceeds the width of the land. When your feed rate exceeds the width of the land, it makes use of the rake angle and chip-forming ability of the insert geometry. The rake angle shears the material rather than the land “rubbing off” the material.
If you are machining with a feed rate that is smaller than the land width, heat develops, reducing tool life.
Optimizing your feed rate and land width also makes use of the chip-forming ability of the geometry. The various angles and “bumps” that make up the geometry are designed to drive the chip into obstructions that can mechanically break the chip. For lower-feed applications, these chip formers, or bumps, need to be close to the edge line, especially at the insert nose radius.
The hardest chip breaking, which creates the shortest chips, occurs just above the nose radius for the cutting depth.
So, adjusting the cutting depth and the nose radius is essential to optimize the cut. Using a smaller nose radius than usual, with a wiper, can be a successful, though less conventional, method. And in some instances, like in the case of extremely tough material, it may be advantageous to break the chip against the primary land. Using a negative angle on the primary land essentially creates a “back wall” out of the chip former on the edge line, which in these unique circumstances, can be the only way to control the chip.
Program for Success
When you are trying to break a chip, proper programming can make all the difference.
A simple example is the common toolpath of an OD turn with an out face. When we out face (face a shoulder by pulling away from the centerline of the part) with a CNMG insert, the lead angle is 85 degrees. This is bigger than a lead angle typically seen on a high-feed milling cutter, which is usually 75 to 80 degrees. The premise of a high-feed milling cutter is to thin the chip out as much as possible to increase productivity by using a feed rate of 0.040 to 0.060 in. per tooth. When the insert is faced out, a long, ribbon-like chip is formed. Instead, a better technique is to in face or face from the OS to the centerline of the part. By doing so we are making use of the -5-degree lead angle and maximizing the chip thickness.
Keep Cool
The advent of high-pressure coolant (HPC) has made an impact on chip control in ductile materials.
Coolant streams at pressures of 1,000 PSI can break titanium chips into what could be compared to a coarse powder. The same technology has been tested in typical automotive steels with no success, and it has been determined that pressures of more than 2,000 PSI are necessary to provide chip control in these materials.
Typically, high-pressure pump companies offer pumps that provide 1,000 PSI with a water-based coolant and 2,000 PSI for straight oil. However, in the near future we will see these companies offering higher-pressure pumps to deal with these problems.
Knowing how to handle these materials effectively by using proper cutting tool parameters, the latest insert geometries, and high-pressure coolants to improve productivity and profitability will help shops keep up with increased demand for automotive transmission components now and in the coming years.
Kevin Burton is product specialist, Sandvik Coromant Canada, 905-826-8900, www.sandvik.coromant.com.
subscribe now
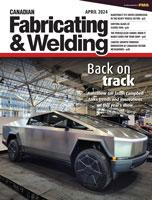
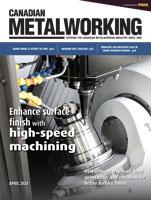
Keep up to date with the latest news, events, and technology for all things metal from our pair of monthly magazines written specifically for Canadian manufacturers!
Start Your Free SubscriptionAbout the Author
- Industry Events
MME Winnipeg
- April 30, 2024
- Winnipeg, ON Canada
CTMA Economic Uncertainty: Helping You Navigate Windsor Seminar
- April 30, 2024
- Windsor, ON Canada
CTMA Economic Uncertainty: Helping You Navigate Kitchener Seminar
- May 2, 2024
- Kitchener, ON Canada
Automate 2024
- May 6 - 9, 2024
- Chicago, IL
ANCA Open House
- May 7 - 8, 2024
- Wixom, MI